Department of Molecular Embryology
Head: Doc. Ing. Petr Dvorak, CSc.
Scientists:
MVDr. Ales Hampl, CSc.
Mgr. Jiri Pachernik, Ph.D.
Ph.D. Students:
Mgr. Vitezslav Bryja
Mgr. Jitka Faitova
Mgr. Jana Kroupova
Technical Assistants:
Olga Lostakova
Stanislava Koskova
Address:
Zemedelska 1, 613 00 Brno
Phone/Fax:(+42) 545 133 298
E-mail: dvorakp@mendelu.cz
The Department of Molecular Embryology is a joint institution with the Laboratory of Molecular Embryology,
Mendel University of Agriculture and Forestry Brno.
Currently, various approaches are being used, including cell and embryo cultures, confocal microscopy, protein analyses, recombinant DNA strate.
gies etc., to address the following topics:
- The functions of fibroblast growth factors (FGFs), their high affinity receptors (FGFRs), and non-signaling coreceptors in early development in mammals
- The role of FGFs and FGFRs in haematopoietic malignanciesbr>
- The functions of cell cycle regulators in female reproductive organs, female germ cells, and cells of embryonal origin
- Developments toward regulatable propagation and differentiation of mouse and human embryonic stem (ES) cells; understanding the molecular mechanisms
that underlie the biological properties of ES cells
The functions of fibroblast growth factors (FGFs), their high affinity receptors (FGFRs), and non-signaling coreceptors in early development in mammals
FGFs constitute an extensive family of signaling molecules with variable functions in development and in adult physiology
and pathology. FGF signals are transmitted via members of the FGF receptor (FGFR) family, of which FGFR-1 and -2 function from the earliest stages of development.
Besides cognate FGFRs, FGFs also interact with non-signaling co-receptors, proteoglycans, that modulate their biological activity.
These FGF co-factors are largely described as heparin-like molecules occuring on the cell surface and in the extracellular matrix, where they may also
serve some regulatory function. In mouse preimplantation embryos, the majority of proteoglycan/embryoglycan carbohydrate ectodomains is nonsulfated and
thus neutral. In this context, we have recently proposed a new concept that these embryoglycan domains, including their shedded ectodomains, could also act
as FGF-2 co.receptors contributing to regulation of mesoderm induction. We have shown that FGF-2 lowaffinity binding function is displayed by an embryoglycan-specific
epitope recognized by TEC-1 antibody that corresponds to LeX trisaccharide Gal(b - 4)-[Fuc(a - 3)]GlcNAc or
its isoforms. When we addressed the potential roles of three naturally occuring isoforms of Lewis X (LeX) in FGF-2-mediated proliferation of
embryonic stem (ES) cells, we have found that (A) O-linked LeX oligosaccharides can regulate the mitogenic activity of FGF.2 in embryonic cells, (B) and this
ability varies with subtle modifications in their structure. Importantly, our data represent the first insight into the mechanism of how growth factor activities might be
modulated by shedded embryoglycan ectodomains.
Each member of the FGFR family interacts with a particular FGF with varying affinity, and this ligand binding specificity of the receptor together
with its downstream signaling diversities, establishes the cellular response. We use targeted disruption of FGFR-1 to interfere with FGF.2 signaling. Using adherent
cultures of nondifferentiated ES cells and ES cell-derived differentiating EBs, we have demonstrated that in ES cells, FGFR-1 plays a dominant role in receptor-ligand
interactions.
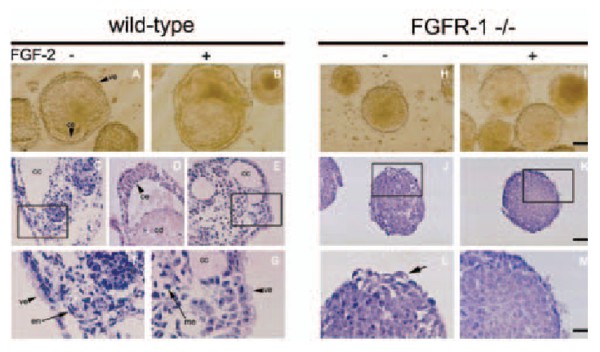
Fig. 1: Morphology of wild-type (left panel) Fibroblast growth factor receptor-1-deficient (right panel)
embryonic stem cell-derived embryoid bodies (EBs)
However, the lack of functional FGFR-1 does not contribute to basal as well as FGF-2-mediated proliferation
of ES cells. Rather, it functions to regulate the differentiation of ES cell-derived EBs. Specifically, we presented evidence that the absence of FGFR-1 causes
down-regulation of endoderm-specific genes. Moreover, it suppresses the formation of the visceral endoderm and the specification of outer layers of the primitive
ectoderm into the columnar epithelium, and thus leads to a block of cavitation of mammalian embryo.
The role of FGFs and FGFRs in haematopoietic malignancies
FGF-2, a prototype member of the heparin-binding growth factor family of FGFs, is a potent mitogen for a
number of cell types. Its potency to stimulate proliferation and migration of endothelial cells has been directly associated with angiogenesis and thus with the growth,
dissemination and metastasis of solid tumors. Recent data suggest that similar phenomenon of FGF-2-induced tumor angiogenesis might take place also in
haematological malignancies. In the past few years, we have performed studies on a large panel of patients suffering from chronic myeloid leukemia (CML) and
revealed increased levels of fibroblast growth factor 2 (FGF-2) in plasma and over-expression of fibroblast growth factor receptor 3 (FGFR-3) in peripheral white
blood cells. Very recently, we found that the vast majority of FGFR-3 signals arise from CD34+ haematopoietic progenitor cells and that the same fraction of
progenitor/stem cells abundantly synthesizes multiple isoforms of FGF-2. Based on these findings, we hypothesize that such growth factor/growth factor receptor
imbalance is of some functional significance in CML. We propose that FGFR-3 might serve a predictive function in patients treated for CML, e.g. by stem cell
therapy or STI571, or might even represent a molecular target(s) for the treatment of CML.

Fig. 2 Localization of the cell cycle regulators in meiotically maturing mouse oocytes.
The functions of cell cycle regulators in female reproductive organs, female germ cells, and cells of embryonal origin
In all multicellular organisms, appropriate progression of ontogenesis depends largely on precise
coordination of cell proliferation and differentiation. The proper coordination of growth- and differentiationrelated processes is ensured by the coordinated
action of cyclin-dependent kinases (CDKs). The mechanisms that evolved to regulate the activity of CDKs include heterodimerization of CDK with a cyclin subunit
to form a holoenzyme, changes in phosphorylation, and interactions with members of a group of regulatory molecules, commonly called CDK inhibitors (CKIs).
It emerged recently that changes in the amount of CKIs during the course of differentiation might also serve some other function(s) besides simply
preventing further cell proliferation via the inhibition of CDKs. We employ several cellular systems to further investigate such functions of cell cycle regulators,
particularly p27 CKI and D-type cyclins, in cell differentiation. First, based on our findings, we recently offered a scenario as to how p27 and cyclin D3 may
participate in driving development and the maintenance of a differentiated phenotype of the luteal cells of the mouse ovary. Second, in studies employing a panel
of embryonal carcinoma (EC) cells that differ from each other by the expression of membrane carbohydrates, we found that the increased amount of p27 protein
observed in differentiating EC cells might also, at least in its early phase, mediate the occurrence of phenomena that are not directly linked to modification of cell
cycle progression. Third, we started investigating whether or not G1/S regulators may play some role in the regulation of the peculiar development of
mammalian female gametes - oocytes. We already described the dynamics of the expression of D-type cyclins, p27, and CDK4 during growth and meiotic
maturation of mouse oocytes. We also characterized the intracellular localization of these molecules and the changes that occur in their phosphorylation status
during the progression of meiotic maturation.
Developments toward regulatable propagation and differentiation of mouse and human embryonic stem (ES) cells; understanding the
molecular mechanisms that underlie the biological properties of ES cells
Mouse embryonic stem cells (ES cells) are immortal cell lines derived from the inner cell mass
of 3.5 day-old blastocysts that can be propagated in vitro in a non-differentiated state in the presence of leukemia inhibitory factor (LIF). When reintroduced
back to the mouse blastocyst, they can give rise to cells of any cell lineage of the developing mouse, including germ line cells. Recently, ES cell lines
were established from many mammalian species, including humans. Due to their properties, ES cells may provide a rich source for in vitro studies, such
as screening for teratogenic and toxic compounds, the identification of gene targets for new drugs or the identification of genes that could be used for
tissue regeneration therapies, and most importantly they may be used for transplantation therapies. In the past few years, strategies have been developed
that allow for the differentiation of ES cells into specific cell lineages, including hematopoietic, myogenic, neural/neuronal, and others. In our laboratory
we concentrate on developing/improving the strate gies that will allow for effective differentiation of ES cells into various neural lineages. Recently, we have
shown that a differentiation protocol involving 2 days of culture in serum-containing media followed by culture in media with only insulin, transferrin,
selenium, and fibronectin, preferentially supports the development of neural cells from mouse ES cells. This differentiation approach may thus
represent a novel alternative to the widely used protocols that invariably employ the formation of embryoid bodies. It is believed that it might serve as an
experimental system for studying the molecular mechanisms involved in neurodifferentiation and also as a basis for further simplifying the generation of neural
cells in vitro.
Relevant publications before 1998
1. Hampl, A., Eppig, J.J. (1995) Analysis of the mechanism(s) of metaphase I arrest in maturing mouse
oocytes. Development 121: 925-933
2. Dvorak, P., Dvorakova, D., Yoshiki, A., Ohashi, T., Kitamura, K., Kusakabe, M. (1995) Expression of paternal
and maternal mitochondrial HSP 70 family, hsc 74, in preimplantation mouse embryos. Int. J. Develop. Biol. 39: 511-517
3. Dvorak, P., Yoshiki, A., Dvorakova, D., Flechon, J.E., Kusakabe, M. (1995) Cell mixing during the early
development of mouse chimera. Int. J. Develop. Biol. 39: 645-652
4. Hampl, A., Eppig, J.J. (1995) Translational regulation of the gradual increase in histone H1 kinase activity
in maturing mouse oocytes. Mol. Reprod. Dev. 40: 9-15
5. Dvorak P., Flechon, J.E., Thompson, E.M., Horak, V., Adenot, P., Renard, J.P. (1997) Embryoglycans regulate
FGF-2-mediated mesoderm induction in the rabbit embryo. J. Cell Sci. 110: 1-10
Publications 1998-2002
1. Dvorak, P., Hampl, A., Jirmanova, L., Pacholikova, J., Kusakabe, M. (1998) Embryoglycan ectodomains
regulate biological activity of FGF-2 to embryonic stem cells. J. Cell. Sci. 111: 2945-2952
2. Eppig, J.J., Hampl, A., Hirao, Y. (1998) Ovarian teratocarcinogenesis: A consequence of abnormal
regulation of meiosis. In: Ovulation:Evolving Scientific and Clinical Concepts, ed. Eli Y Adashi, Springer Verlag
3. Oh, B., Hampl, A., Eppig, J.J., Solter, D., Knowles, B.B. (1998) SPIN, A substrate in the MAP kinase
pathway in mouse oocytes. Mol. Reprod. Dev. 50: 240-249
4. Jirmanova, L., Pacholikova, J., Krejci, P., Hampl, A., Dvorak, P. (1999) O-linked carbohydrates are
required for FGF-2-mediated proliferation of mouse embryonic cells. Int. J. Develop. Biol. 43: 555-562
5. Hampl, A., Pachernik, J., Dvorak, P. (2000) Levels and interactions of p27, cyclin D3, and CDK4 during the
formation and maintenance of the corpus luteum in mice. Biol. Reprod. 62: 1393-1401
6. Krejci, P., Dvorakova, D., Krahulcova, E., Pachernik, J., Mayer, J., Hampl, A., Dvoøak, P. (2001) FGF-2
abnormalities in B-cell chronic lymphocytic and chronic myeloid leukemias. Leukemia, 15: 228-237
7. Krejci, P., Dvorakova, D., Krahulcova, E., Hampl, A., Dvorak, P. (2000) Fibroblast growth factor 2 (FGF-2)
and leukemia. What might be the significance of the increased FGF-2 synthesis for leukemia cells? Scripta Medica (Brno) 73: 142
8. Dvorakova, D., Krejci, P., Mayer, J., Fajkus, J., Hampl, A., Dvorak, P. (2001) Changes in the expression of
FGFR3 in patients with chronic myeloid leukaemia receiving allogeneic peripheral blood stem cell transplants. Brit. J. Haematol. 113: 832-835
9. Dvorak, P., Campion, E., Flechon, J.E., Hampl, A., Renard, J.P. (2001) Modulation of protein synthesis in
rabbit ICM-derived cells by FGF2. Reprod. Nutr. Dev. 41: 197-205
10. Pachernik, J., Soucek, K., Hampl, A., Hofmanova, J., Kozubik, A. (2001) Transforming growth factor-ß1
induces junB mRNA accumulation, G1-phase arrest, and pRb dephosphorylation in human leukemia HL.60 cells. Folia Biologica (Praha) 47: 32-35
11. Sutovsky, P., Motlik, J., Neuber, E., Pavlok, A., Schatten, G., Palecek, J., Hyttel, P., Adebayo, O.T., Adwan,
K., Alberio, R., Bagis, H., Bataineh, Z., Bjerregaard, B., Bodo, S., Bryja, V., Carrington, M., Couf, M., de la
Fuente, R., Diblik, J., Esner, M., Forejt, J., Fulka, J. Jr., Geussova, G., Gjorret, J.O., Libik, M., Hampl, A.,
Hassane, M.S., Houshmand, M., Hozak, P., Jezova, M., Kania, G., Kanka, J., Kandil, O.M., Kishimoto, T.,
Klima, J., Kohoutek, J., Kopska, T., Kubelka, M., Lapathitis, G., Laurincik, J., Lefevre, B., Mihalik, J.,
Novakova, M., Oko, R., Omelka, R., Owiny, D., Pachernik, J., Pacholikova, J., Peknicova, J., Pesty, A.,
Ponya, Z., Preclikova, H., Sloskova, A., Svoboda, P., Strejcek, F., Toth, S., Tepla, O., Valdivia, M., Vodicka,
P., Zudova, D. (2001) Accumulation of the proteolytic marker peptide ubiquitin in the trophoblast of mammalian blastocysts.
Cloning Stem Cells 3: 157-161
12. Pachernik, J., Hampl, A., Soucek, K., Kovarikova, M., Andrysik, Z., Hofmanova, J., Kozubik, A. (2002)
Multiple biological effects of inhibitors of arachidonic acid metabolism on human keratinocytes. Arch. Dermatol. Res. 293: 626-633
13. Esner, M., Pachernik, J., Hampl, A., Dvorak, P. (2002) Targeted disruption of fibroblast growth factor
receptor 1 blocks maturation of visceral endoderm and cavitation in embryoid bodies. Int. J. Dev. Biol. 46: 817-825
14. Pachernik, J., Esner, M., Bryja, V., Dvorak, P., Hamp,l A. (2002) Neural differentiation of mouse embryonic
stem cells grown in monolayer. Reprod. Nutr. Dev. 42: 317-326
15. Preclikova, H., Bryja, V., Pachernik, J., Dvorak, P., Hampl, A. (2002) Early cycling-independent changes to
p27, cyclin D2, and cyclin D3 in differentiating mouse embryonal carcinoma cells. Cell Growth & Differ. 13: 421-430
16. Horak, D., Dvorak, P., Hampl, A., Slouf, M. (2003) Poly(2-hydroxyethyl methacrylate-co-ethylene
dimethacrylate) as a mouse embryonic stem cells support. J. Appl. Polymer Sci. 87: 425-432
17. Krejci, P., Faitova, J., Laurell, H., Hampl, A., Dvorak, P. (2003) FGF-2 expression and its action in human
leukemia and lymphoma cell lines. Leukemia 17: 818-820
18. Anger, M., Kues, W.A., Klima, J., Mielenz, M., Kubelka, M., Motlik, J., Esner, M., Dvorak, P., Carnwath,
J.W., Niemann, H. (in press) Cell cycle dependent expression of PLK 1 in synchronized porcine fetal fibroblasts. Mol. Reprod. Dev.
19. Anger, M., Bryja, V., Jirmanova, L., Hampl, A., Carrington, M., Motlik, J., Dvorak, P., Kubelka, M. (2003)
The appearance of truncated cyclin A2 correlates with differentiation of mouse embryonic stem cells. Biophys. Biochem. Res. Com., 32: 825-830.
|